Practice Essentials
Brain injury is often defined differently in published reports. Although many authors use the term brain injury to mean acute traumatic damage to the central nervous system (CNS), others use the term head injury, which allows inclusion of skull injuries, fractures, or soft tissue damage to the face or head without any obvious neurologic consequences. Brain contusions commonly are identified in patients with traumatic brain injury (TBI) and represent regions of primary neuronal and vascular injury. [1] Contusions are formed in 2 ways: direct trauma and acceleration/deceleration injury. [2, 3, 4]
TBIs may be closed or open injuries and are categorized on the basis of severity as measured by the Glasgow Coma Scale score (GCS). A score of 15 suggests normal functioning, whereas a score of 3 indicates unresponsiveness. Patients with severe TBIs have a GCS of 8 or less; 9-12 is moderate; and mild is 13-15. [5]
Contusions of the brain are most commonly seen in the inferior frontal lobe and the anterior inferior temporal lobe. During the first 48 hours, they can significantly increase in size, which is referred to as blooming. Progression of cerebral contusions occurs in up to 75% of patients with TBI. Other sites can be affected through a coup (beneath the impact) or contrecoup (opposite to impact).
Computed Tomography
CT scanning is an excellent modality for defining contusions. Contusions often are not appreciated on the first CT scan obtained immediately after trauma, but they become obvious on follow-up scans. Initially, the false-negative rate is high, but false-positive findings are negligible.
Contusions may progress with time. Imaging findings in brain contusions tend to vary because of the stages of evolution common to these lesions.Acute CT scans initially demonstrate isoattenuating contusions that become more evident on follow-up CT scanning. CT scans, as shown below, often demonstrate progression over time in the size and number of contusions and the amount of hemorrhage in the contusions. Initially, CT scan findings can be normal or minimally abnormal because the partial volumes between the dense microhemorrhages and the hypodense edema can render contusions isoattenuating relative to the surrounding brain tissue. [21, 22, 23]
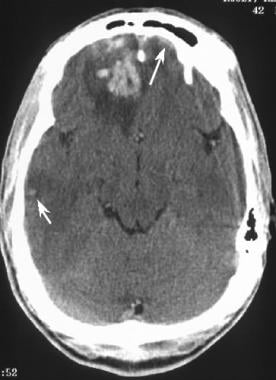
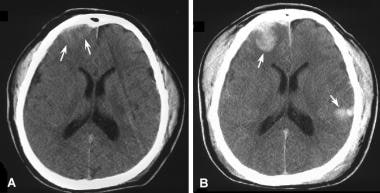
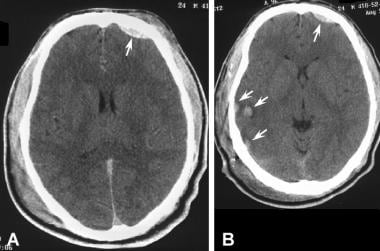
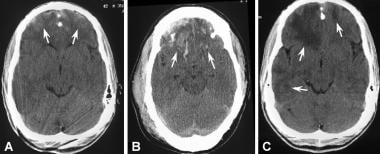
Gliding contusions (examples of which appear in the image below) are due to sagittal angular acceleration with stretching and tearing of the parasagittal veins. Gliding contusions are often hemorrhagic, not only from the differential motion of subcortical structures (commonly referred to as shear injury), but also from tearing of parasagittal veins. When the brain abruptly shifts at the time of impact, the subcortical tissues glide more than the cortex. The convexities of each hemisphere are anchored to the dura by arachnoid granulations. Gliding contusions also tend to be bilateral.
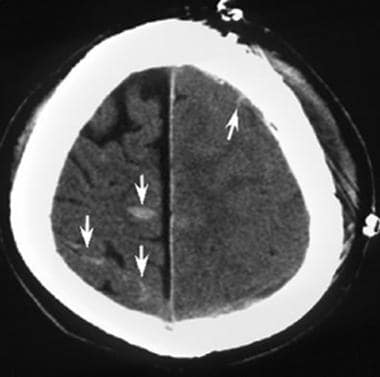
The image below shows a CT scan compared to a xenon blood-flow image. On CT scan, the contusions are seen in the bifrontal regions as hyperintense areas. The corresponding xenon blood-flow image shows dark regions that indicate decreased perfusion in the contused areas of the brain.
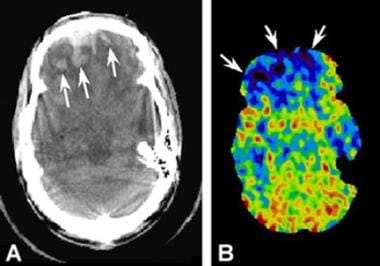
The image below shows CT and MRI scans of acute contusions.
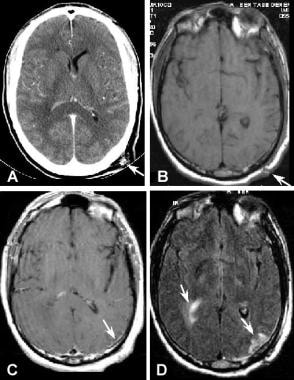
Magnetic Resonance Imaging
MRI is the criterion standard for defining contusions, and scans typically demonstrate brain contusions from the onset of injury. It is sensitive but nonspecific for brain injuries. Ischemic lesions often depicted in the normal aging brain may be difficult to distinguish from traumatic injuries in the acute phase. MRI is sensitive to hyperacute hemorrhagic contusions (< 12 hr). On MRI, contusions are isointense to hyperintense on T1-weighted images and hyperintense on T2-weighted images, as shown in the images below. Gradient-echo MRIs may reveal hypointensity, which is critical to the detection and delineation of contusions. [32]
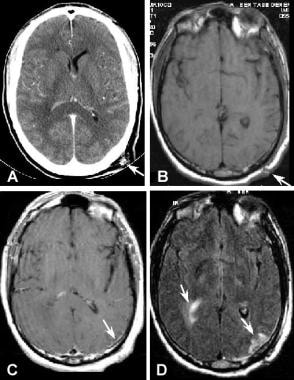
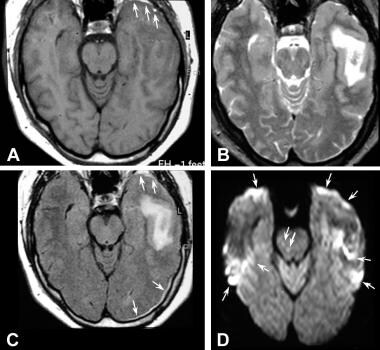
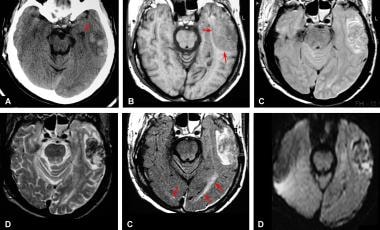
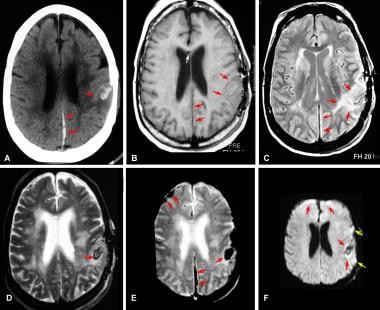
Use of fast fluid-attenuated inversion recovery (FLAIR) sequences has made detection of accompanying subarachnoid hemorrhage possible, with a sensitivity that is equal to or greater than that of CT. Using FLAIR sequences, subarachnoid hemorrhage produces dramatic hyperintensity in the normally hypointense cerebrospinal fluid. Additionally, companion FLAIR images show the extent of contusions better than most traditional MRIs. Degree of confidence is excellent with FLAIR imaging.
Use of diffusion-weighted imaging (DWI) in acute brain trauma has not been described in depth in the literature. DWI allows the rapid detection of an ischemic region after the onset of brain injury. The signal intensity is increased in the affected region on DWIs. DWIs show areas of restricted diffusion in areas associated with cerebral contusions.
Akiyami et al reported that susceptibility-weighted MRI can be very sensitive in visualizing and detecting microhemorrhages. In their study, they found that susceptibility-weighted MRI detected a mean of 76 ± 52 (total, 1132) hypointense spotty lesions and that T2-weighted images detected a mean of 21 ± 19 (total, 316) lesions. [33]
Gadolinium-based contrast agents have been linked to the development of nephrogenic systemic fibrosis (NSF) or nephrogenic fibrosing dermopathy (NFD). The disease has occurred in patients with moderate to end-stage renal disease after being given a gadolinium-based contrast agent to enhance MRI or magnetic resonance angiography (MRA) scans. NSF/NFD is a debilitating and sometimes fatal disease. Characteristics include red or dark patches on the skin; burning, itching, swelling, hardening, and tightening of the skin; yellow spots on the whites of the eyes; joint stiffness with difficulty moving or straightening the arms, hands, legs, or feet; pain deep in the hip bones or ribs; and muscle weakness.
Nuclear Imaging
Currently, functional imaging is not widely used in TBI assessment. Umile et al noted that single-photon emission computed tomography (SPECT) blood-flow imaging with technetium-99m (99mTc) hexamethylpropyleneamine oxime (HMPAO) uptake is sensitive enough to detect diffuse changes in patients with decreased blood flow due to depression. Many studies of mild, moderate, and severe TBI in the acute, subacute, and chronic stages have shown that SPECT is more sensitive than CT and MRI, and scans can depict changes even when findings are normal. [34] Comparisons consistently show that in mild cases of TBI, SPECT scans identify more brain abnormalities than conventional CT scanning or MRI. SPECT findings are particularly sensitive in patients with mild postconcussive symptoms. SPECT scans can depict focal changes in 53% of patients with mild head injury who showed few abnormal findings on MRI and CT scans. [35]
SPECT results are correlated with the severity of injury. A negative finding on SPECT in the first 4 weeks is predictive of a good outcome. SPECT findings also can help in predicting a poor outcome, posttraumatic headaches, and clinical deterioration in patients with intracerebral hematomas. Studies correlating SPECT with neuropsychologic testing have been inconsistent. One study reported that SPECT can reveal significant increases in blood flow following cognitive rehabilitation therapy 2 years after TBI; these findings were correlated with improvements on neuropsychological tests. [36]
It is unclear whether positron emission tomography (PET) scanning is more useful than MRI or CT scanning. PET images are more anatomically detailed than SPECT images. Limitations to the use of PET scanning are the time required to complete the images, the cost, and the lack of widespread availability. Scans require from 2 to 40 minutes to complete. Also, not all facilities have access to PET scanners. [2, 37]
Xenon is an inert noble gas that is diffusible across the blood-brain barrier. The inhalation of nonradioactive xenon can be used to calculate physiologic aspects of brain function, including regional cerebral blood flow, by using CT scanning. Xenon imaging has been used for the documentation of brain death. Brain trauma is a potential application for xenon CT scanning.
Xenon gas is expensive; without a rebreathing apparatus, approximately 10 L of xenon is required for each study, adding additional costs to each examination. Also, anesthetic equipment must be used, and special software must be purchased for the CT scanner. The anesthetic effects of xenon are somewhat worrisome as well. Multiple, rapid-sequence images must be obtained at the same level, limiting the total number of section levels that can be obtained. This factor may limit the scan to a few regions, when a given patient may have multiple pathologic areas.
Caution must be taken not to mistakenly attribute the diffuse changes seen in psychiatric disorders with focal TBI changes.
Angiography
Focal vascular spasm may be seen in the acute/subacute injury phase. Spasm is a good indicator of subarachnoid hemorrhage and underlying brain injury. Few false-positive findings occur, but angiography may not reveal the true extent of injury. Traumatic aneurysms are rare but may occur as a result of either blunt or penetrating brain trauma. Intracranial aneurysms may result from fracture, with laceration of the adjacent artery by bone spicules or by shearing forces secondary to rapid deceleration injury. Penetrating wounds, usually from bullets or shrapnel, are another etiology. Most traumatic aneurysms are pseudoaneurysms, meaning that they are hematoma cavities contained by the soft tissues adjacent to an arterial laceration. As the hematoma organizes and resolves, it becomes surrounded by a fibrous pseudocapsule, which is highly prone to rupture.
Cerebral aneurysms are potentially catastrophic lesions, and many persons who do survive aneurysm rupture are permanently disabled. Treatment of unruptured aneurysms is associated with low morbidity and mortality, which makes accurate and timely diagnosis important.
Conventional angiography remains the definitive procedure for the preoperative evaluation of aneurysms. However, the cross-sectional imaging modalities, including computed tomography angiography and MRA, add indispensable information concerning the size of the lesion, location, presence of thrombus, associated hemorrhage, and condition of surrounding brain tissue.
-
Schematic diagrams of contusion locations in sagittal midline (A), lateral (B), and base (C) views show the areas most commonly affected by contusions (red) and those that are occasionally affected by contusions (blue). Areas that are predominantly affected by contusions include the orbitofrontal cortex, anterior temporal lobe, and posterior portion of the superior temporal gyrus area, with the adjacent parietal opercular area. Areas that are less commonly affected include the lateral midbrain, inferior cerebellum and adjacent tonsil, and the midline superior cerebral cortex.
-
Acute brain contusion. Axial CT scan obtained in a patient immediately after a high-speed motor vehicle accident demonstrates a large, right frontal contusion with hemorrhage and surrounding edema. A smaller, subtle, right temporal cortical contusion (short arrow) is noted, as well as a small, left frontal subdural hematoma (long arrow).
-
Enlargement of an acute brain contusion. (A) Axial CT scan obtained on day 1 after head trauma shows a subtle area of slightly hypoattenuating right frontal lobe contusion (arrows) with a small, overlying, right frontal subdural hematoma. Note that the scalp hematomas in the frontal and temporal regions indicate areas of direct force. (B) Companion axial CT scan obtained on day 2 shows a large, right frontal contusion (arrow) and a new, large, left temporal contusion (arrow). Note that the scalp hematomas have increased in size.
-
Evolution of an acute brain contusion. (A) Axial CT scan obtained immediately following severe blunt trauma to the head shows a small, left frontal epidural hematoma (arrow). Extensive subgaleal bicranial hematomas are seen. (B) Companion CT scan obtained 6 days after trauma shows the small, left frontal epidural hematoma (long arrow) and smaller areas of subgaleal bicranial hematomas. Note that the previously isoattenuating contusion in the right posterior temporal area is now evident (short arrows in B).
-
Resolution of a brain contusion. (A) Axial CT scan obtained on day 1 after a high-speed motor vehicle accident shows subtle evidence of bifrontal hemorrhagic contusions (arrows). (B) Axial CT scan obtained on day 2 shows increased hemorrhage within the inferior frontal cortex bilaterally (arrows). (C) Axial CT scan obtained on day 14 shows resolution of the bright blood (upper arrows) and residual areas of dark edema in both frontal lobes and a subtle area throughout the right temporal lobe (lower arrows).
-
Acute gliding brain contusions. Axial CT scan obtained immediately after blunt trauma to the left convexity of the skull resulted in severe swelling of the entire left cerebral hemisphere with loss of the gyral pattern secondary to edema. A small collection of subarachnoid blood is present (up arrow). The right hemisphere shows contrecoup gliding contusions (down arrows).
-
Comparison of a CT scan with a xenon blood-flow radionuclide scan. (A) CT scan shows bifrontal contusions following severe head trauma (arrows). (B) Companion CT scan showing xenon uptake demonstrates dark regions (arrows), indicating decreased perfusion in the contused brain.
-
Comparison of a CT scan and MRIs showing acute contusions. (A) Contrast-enhanced axial CT scan obtained immediately after head trauma shows a foreign body in the scalp (arrow) that marks the site of direct impact. Blood is noted in the right lateral ventricle. Chronic white-matter changes are present. (B) Axial T1-weighted MRI obtained on the same day shows the scalp changes at the site of the trauma (arrow). There is minimal underlying superficial cortical hyperdensity consistent with cortical contusion. (C) Comparison gadolinium-enhanced T1-weighted MRI shows minimal enhancement of the left posterior temporal-occipital cortex below the site of the scalp trauma (arrow). (D) Companion fluid-attenuated inversion recovery (FLAIR) MRI shows the extent of the left cortical contusion (arrow). Note the hyperintense signal from blood in cerebrospinal fluid and the displaced right lateral ventricle with surrounding signal intensity changes in the adjacent optic radiations (arrow).
-
MRI in a 2-day-old brain contusion. (A) T1-weighted image shows obliteration of the left temporal horn due to subdural hemorrhage (arrows). (B) T2-weighted and (C) fluid-attenuated inversion recovery (FLAIR) images show hyperintense signal surrounding isointense blood in the left temporal lobe. The FLAIR image shows the left anterior temporal subdural hemorrhage and a posterior extension more clearly (arrow). (D) Diffusion-weighted MRI shows areas of restricted diffusion in the left temporal lobes and 2, small, abnormal areas in the right midbrain (arrows). The bilateral anterior frontal and temporal hyperintense areas represent artifacts (arrows).
-
Comparison of a CT scan and MRIs obtained 1 day after acute trauma. (A) Acute CT scan shows a large, temporal-lobe contusion lateral to the displaced left temporal horn (arrows). (B) T1-weighted MRI shows hypointense signal in the left temporal lobe, with mass effect causing a clear loss of sulcal pattern (arrows). (C) Spin-density MRI shows mixed signals within the contusion with predominant isointensity. Note partial volume artifacts surrounding the brainstem.(D) Spin-echo T2-weighted MRI shows mixed intensity throughout the large left temporal contusion. (E) Fluid-attenuated inversion recovery (FLAIR) MRI demonstrates petechial hemorrhages that are isointense to the brain. Note the accompanying abnormally bright signal in the left optic radiations (arrows); one of many small shearing injuries is seen at the right occipital cortex/gray matter junction (arrow). (F) Diffusion-weighted MRI shows mixed signals with restricted diffusion in the most posterior aspect of the large left temporal contusion. An artifact appears in the right temporal lobe.
-
Comparison of a CT scan and MRIs obtained 4 hours after acute trauma. (A) CT scan shows focal contusion in the left superior temporal gyrus (arrow) and a posterior falx subdural hematoma (arrows). Incidental white matter changes are present in both hemispheres. Incidental left parietal bone postsurgical changes are present. (B) T1-weighted MRI shows slight hypointensity in the contusion (arrows) and isointense signal in the subdural (arrows). Incidentally noted in the anterior corpus callosum is a small, hypointense scar from a prior intraventricular shunt (white arrow). Postsurgical changes in the left parietal bone are depicted. (C) Spin-density MRI shows a large area of hyperintense signal at the contusion site (arrows) and hyperintensity in the subdural blood (arrows).(D) T2-weighted MRI shows hyperintense edema surrounding the hemorrhagic area, which has a hypointense ring (arrow) and an isointense center. The subdural blood is inconspicuous on this image. Chronic white-matter signal abnormalities match those seen on the CT scan (A). (E) Gradient-echo MRI shows marked hypointense signal in the falcine subdural hematoma (arrows), contusion (arrow), and right frontal region, representing magnetic susceptibility products due to subacute hemorrhage. (F) Diffusion-weighted MRI shows restricted diffusion in a zone surrounding the contusion (arrows). Artifacts are depicted in the cortex of both hemispheres (red and yellow arrows).